Exploring the Value of Vehicle to Grid (V2G) for California
This document, prepared by Evolved Energy Research, investigates the benefits and implications of Vehicle to Grid (V2G) technology in California as of March 2025.
Exploring the Value of Vehicle to Grid (V2G) for California Prepared by: EER March 2025
page 2 • UCS partnered with Evolved Energy Research (EER) to develop a study on the incremental benefits from different scales of light-duty vehicle-grid integration (VGI) in the context of California’s decarbonizing energy system. UCS has focused on California because of its policy goals and the large number of projected light-duty EVs in the state that could represent a significant flexibility resource for the grid. • EER used its RIO model, adapting the least-cost decarbonization scenario from EER’s 2024 Annual Decarbonization Perspective to assess the long-term benefits of VGI. RIO quantifies both electricity generation cost and electricity transmission and distribution (T&D) costs by tracking peak load across representative distribution circuits, known as ‘feeders.’ For this project, EER developed novel model capabilities to enable constrained optimization of charging and discharging behavior across unique feeder charging shapes, including costs for deviating vehicle state of charge from its target level. • The goal of the modeling is to articulate how the value of VGI changes as the scale and capabilities of optimized charging and discharging increase over time. The analysis explores ranges of customer participation to illustrate how benefits change. • Results from the capacity expansion modeling focus on how VGI enables lower system costs and the relative benefits of different types and scales of VGI by 2045. • Various California Energy Commission staff working on VGI topics provided valuable feedback on this study's analytical approach and preliminary findings. Study: Partners and purpose
page 3 1. Executive summary 2. Scenario design and assumptions 3. Modeling results • California’s decarbonized energy system • Savings and impact of VGI 4. Looking forward 5. Appendix A – Methodology 6. Appendix B – Supplemental results Table of contents
page 4 • Battery Degradation: The gradual reduction in a battery’s capacity due to charging and discharging cycles. V2G participation may slightly accelerate battery wear but can be managed through proper incentives. • Bulk System Costs: The total expenses related to electricity generation, transmission, and large-scale storage, which VGI can help reduce by optimizing charging behavior and potentially discharging. • California Energy Commission (CEC): The state agency responsible for energy planning, research, and policy development. The CEC oversees a number of VGI programs along with related infrastructure funding and charging standards. • Charging Profile: A pattern of when and how an EV charges, often influenced by TOU rates, driver behavior, and grid conditions. • Constrained Optimization: A modeling approach that optimizes charging and discharging while considering grid limitations, customer preferences, and cost trade-offs. • EnergyPATHWAYS: A demand-side stock-rollover model used to project energy demand and decarbonization pathways based on technology adoption and policy changes. • Feeder: A distribution circuit that delivers electricity from substations to end users. In this study, different feeder types (residential, commercial, industrial) are analyzed for their impact on VGI. • Load Shifting: A strategy where EV charging is delayed or advanced to match grid needs, reducing peak demand and making better use of renewable energy. Glossary of key terms
page 5 • Renewable Energy Curtailment: When excess solar or wind energy cannot be used or stored, leading to wasted generation. VGI can help by charging EVs when renewables are abundant. • RIO (Regional Investment Optimization Model): A macro-energy supply model that determines least-cost investment and operation plans across electricity, fuels, and storage while optimizing for emissions reductions. • Smart Charging (V1G): A one-way charging method that schedules EV charging based on grid conditions, electricity prices, or renewable energy availability to reduce costs and support grid stability. • State of Charge (SoC): The battery charge level of an EV, expressed as a percentage of its total capacity. • Time-of-Use (TOU) Rates: A pricing structure where electricity costs vary depending on the time of day, encouraging users to charge EVs during off-peak hours when energy is cheaper. • Vehicle-Grid Integration (VGI): A broad term for technologies and strategies that allow electric vehicles (EVs) to interact with the grid, optimizing charging and potentially discharging power back to the grid. • Vehicle-to-Grid (V2G): A two-way charging technology where EVs not only charge from the grid but can also discharge power back to support grid stability and reduce system costs. • Vehicle-to-Home (V2H) / Vehicle-to-Building (V2B): The ability of EVs to provide backup power to homes or buildings, improving resilience during outages. Glossary of key terms
1. Executive Summary
page 7 • California has one of the most ambitious light-duty vehicle electrification goals in the country, and EVs are projected to grow significantly to support the state’s goal of carbon neutrality by 2045. This study considers how that investment can help accelerate other aspects of California’s energy transition and reduce the cost of decarbonization in 2045. • An NREL survey1 of studies of the value of managed EV charging suggests large-scale EV adoption can enable flexibility and drive meaningful savings, greater than $1000 per vehicle per year in avoided bulk system investments alone. • National trends show that the costs of distribution systems are rising and have become the largest category of capital spending among grid infrastructure2. EV charging has the potential to either accelerate or mitigate distribution peak demand that drives new distribution investments. This study finds that VGI has the potential to reduce residential peak demand by more than 25% in 2045. • Residential electric rates in California have been outpacing inflation in recent years and are projected to continue to rise.3 The evolution of EV charging will play a significant role in how loads from electric vehicles impact rates. Why study EV charging for California? 1. https://pubs.rsc.org/en/content/getauthorversionpdf/D1EE02206G 2. https://www.eia.gov/todayinenergy/detail.php?id=63724 3. https://www.cpuc.ca.gov/-/media/cpuc-website/divisions/office-of-governmental-affairs-division/reports/2024/2024-sb-695-report.pdf
page 8 • Current time-of-use (TOU) rates are insufficient at driving EV charging behavior to benefit ratepayers. • Current charging patterns combined with California’s electrification ambitions will increase the simultaneous peak of residential customers by more than 50%. • True smart charging with hourly scheduling (V1G1) results in ~$500/year in system benefits per participating vehicle. • Optimal charging patterns show significant variation between days and between different parts of California. • Deployment of vehicle-to-grid (V2G2) technology increases benefits to $900-1,300/year per vehicle. • The marginal value of V2G participation declines significantly above 25% participation of eligible load. • Occasional V2G discharge supports reliability rather than competing with grid-tied battery storage as a daily load-shifting resource. • 90% of the value comes from less than 30% of V2G discharge (less than 600 miles on the battery annually). Dispatching V2G while ignoring associated costs and state-of-charge preferences raises savings by $150/year per vehicle but increases equivalent annual mileage to 2000. • Much of the V2G value is contingent on deferring residential distribution system upgrades with targeted calls for discharge, increasing the demands for information/scheduling. Summary of key insights from the study 1. V1G is a system where EV charging is optimized and controlled to manage grid demand, but energy is not fed back into the grid. 2. V2G is a system where EVs can both charge from and discharge energy back to the grid.
page 9 Implications from the study’s findings • Utilities: VGI can significantly reduce distribution-level peak demand—by over 25% for residential customers by 2045—lowering distribution costs and mitigating rate increases that would occur without VGI. • Regulators: To maximize benefits, VGI must be fully integrated into resource and distribution planning, and early investments are needed to support large- scale deployment. • Investors: The long-run value of V2G lies in displacing reliability resources; successful investments will integrate software, hardware, and program design to enable this reliability role. • Policymakers: VGI is vital for the long-term success of California’s energy policy by controlling costs. Early investments in the technology through expanded pilots, additional incentives, and R&D will pay dividends for California ratepayers.
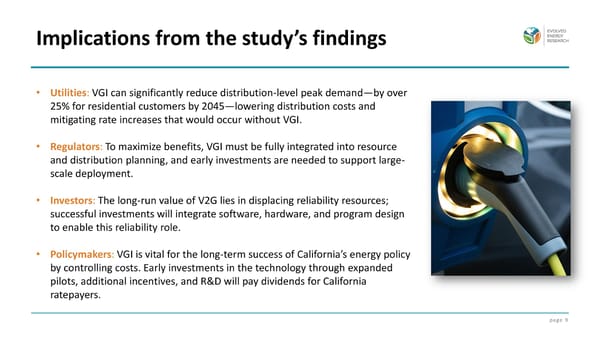
This is a modal window.
page 10 • Optimized charging adjusts to system conditions to minimize costs of infrastructure and generation. • Smart charging is used to align daily charging patterns with grid demands. • Charging behavior is more variable than bulk battery storage, which consistently shifts solar production to evening hours. • VGI reduces the need for bulk storage, especially with V2G participation. VGI complements grid storage operations to increase system flexibility. • V2G discharge is used in hours with reliability needs, supporting the system and displacing more costly reliability resources (e.g., long-duration storage). • For a scenario with 50% V2G participation, 60 days with V2G discharge account for 80% of energy discharged in a year. Only a handful of days utilize more than 60% of the available discharge capability in a key hour. The remaining 20% of energy is spread over 100 more days with low utilization (roughly 10% of capacity). Optimized charging patterns vary daily, and V2G discharge focuses on supporting reliability needs
page 11 • VGI deployment in California saves $2 - $12 billion of annual energy system costs in 2045 (1% - 5% of $240B total energy system costs). • For the same level of VGI, V2G achieves higher savings than V1G by achieving deeper reductions in distribution costs (lower peak demand) and avoiding more storage and generation costs. • Annual savings per participating vehicle in 2045 vary, with the highest per participating vehicle savings in the 20% V2G scenario (over $1,340) and the lowest in the 80% V1G scenario ($470). Savings per vehicle decline with higher VGI participation due to the reduced marginal impact per vehicle. VGI lowers costs, V2G yields the highest savings
2. Scenario Design and Assumptions
page 13 • In 2024, California Senate Bill 59 was signed into law, enabling the California Energy Commission (CEC) to develop requirements for bidirectional charging, which would include the scope, timing, and technical details.1 • CA has many charging programs underway: • PG&E has a pilot vehicle to everything (V2X) program, focused on bidirectional charging for Ford F-150 lighting trucks for residential, electric school buses for commercial, and microgrids. SDGE is also conducting a similar school bus V2G pilot program. • BMW piloted its smart charging program, ChargeForward, in PG&E territory and currently offers the program for eligible vehicles • State agencies are exploring VGI-related topics: • California Public Utilities Commission launched an annual VGI forum in 2024, building on work the agency did to look for strategies to maximize VGI deployment in 2020 • The CEC has a Vehicle-Grid Integration Program, which has previously looked at several topics around charging infrastructure and conducted solicitations on various VGI-related topics. California VGI policy 1. https://www.utilitydive.com/news/california-electric-vehicle-ev-to-grid-battery-capacity/726319/?form=MG0AV3
page 14 • Scenarios explore different levels of enhanced VGI deployment for light- duty EVs: • Existing charging follows the “TOU only” charging shape, with no ability to delay or advance EV charging • V1G capabilities allow vehicles to advance or delay to optimize charging behavior for feeder and system needs • V2G expands V1G to allow the ability to discharge to the grid • The sweep of scenarios looks at 3 levels of VGI and a “base case” scenario, varying the level of V1G and V2G EV charging in each scenario Scenarios assess how VGI’s scale impacts savings TOU only V1G V2G Base 100% 0% 0% 20% VGI 80% 20% 0% 80% 10% 10% 80% 0% 20% 50% VGI 50% 50% 0% 50% 12.5% 37.5% 50% 25% 25% 50% 37.5% 12.5% 50% 0% 50% 80% VGI 20% 80% 0% 20% 66.7% 13.3% 20% 53.3% 26.7% 20% 40% 40% 20% 26.7% 53.3% 20% 13.3% 66.7% 20% 0% 80% 1. LDV EV load available for VGI only excludes DC fast charging loads, which by design are inflexible, and level 1 charging which is too slow to enable meaningful charging flexibility Scenarios by share of LDV load available for VGI1
page 15 • EER modeled four archetypal feeders – Residential, Commercial, Industrial, and DC Fast Charging – to better understand the potential impacts of increasing VGI deployment • The shapes of other non-LD EV loads are assigned by feeder type, e.g., the shape of residential load (HVAC, lighting, water heating) as compared to the shape of industrial load (motors, heavy equipment, etc.) • For the analysis, EER developed LD EV charging shapes by archetypal feeder and charging power level for California by adapting CEC charging shapes. • LD EVs are mapped to feeders with shares of 64% residential, 17% commercial, 3% industrial, and 16% DC fast charging. Level 1 charging represents slightly more than 25% of all charging demand, principally on the residential feeder. • Level 1 charging loads and loads on the DC fast charging are assumed to be unavailable for VGI participation. • The sweep of scenarios explores a broad range of future pathways that allow for different levels of VGI which could be driven by utility programs, direct payments, vehicle manufacturer programs, regulatory requirements, or any number of potential interventions. The feeder archetype structure differentiates both the scale of VGI load as well as charging/discharging behavior could lower system costs (e.g., industrial charging loads are small but generally coincident with solar production). Feeder archetypes differentiate the impacts of VGI depending on where vehicles charge
page 16 • The base scenario adapted light-duty EV charging shapes used by the CEC, including in the 2024 Integrated Energy Policy. The CEC shapes reflect residential charging shapes that are dominated by TOU rates, with charging increasing significantly overnight to take advantage of the lowest rates. • EER smoothed some peaky charging shape behavior to better reflect some form of randomized charging start times, which is a very low-cost measure to limit needle peak charging behavior when very large portions of vehicles start charging the minute the lowest-rate TOU period begins. • The CEC charging profiles were initially developed in 20191, and CEC analyses assume high levels of workplace charging.2 The CEC is working on updating shapes with metered load data, but that process is still ongoing. EER used underlying feeder-level shapes combined with assumptions about relative shares of EV charging load by feeder (on the previous slide) distinct from CEC assumptions. • Shapes vary by feeder and charging level to differentiate behavior and VGI capabilities. • Workplace charging on commercial and industrial feeders reflects when vehicles are at those locations and charging. Relatedly, lower residential charging during the middle of the day reflects fewer vehicles at those locations. The base scenario reflects EV charging shapes that are heavily Time-of-Use influenced 1. Limited documentation is available for the CEC EV charging profile; this 2019 report describes the data used to develop the charging shape and value for previous iterations of the shape. 2. See this CEC presentation for an overview of more recent workplace charging assumptions.
page 17 • EER models VGI by segmenting the participating LDV fleet and modeling optimal charge and potential discharge for each feeder. • This simplification doesn’t capture vehicles traveling between feeders to charge, but it is a reasonable approximation of a large population of vehicles and ensures that the model reflects the required levels of charging at each feeder. • VGI technologies leverage the storage capacity of vehicles to delay or advance charging and potentially discharge storage and send power to the grid. • Optimized charging is constrained by a maximum charging shape (e.g., charging allowed in the daytime on the commercial feeder but not at night) and must be able to meet daily energy needs with the shifted charging behavior. • V2G optimizes charging as with V1G and allows discharging, incurring a round-trip efficiency penalty along with a variable O&M cost based on the estimated useful lifecycle of a vehicle battery.1 • Both V1G and V2G incur a penalty for deviating from a target state of charge, reflecting the amount vehicle owners would need to receive to behave in the system’s optimal manner rather than charging on the assumed existing charging shape. VGI methodology overview State of Charge Charge V2G Discharge Electricity Residential Daily Energy Needs Commercial Industrial State of Charge Charge V2G Discharge State of Charge Charge V2G Discharge Daily Energy Needs Daily Energy Needs 1. See Appendix A for additional information on these assumptions and their derivation
page 18 • Vehicle projections and electricity demand are adapted from the central scenario of EER’s Annual Decarbonization Perspective 2024, calibrated to 2023 California vehicle sales • EV growth rapidly accelerates in California, with 6 million vehicles on the road in 2030, 24 million in 2040, and 36 million in 2050 • EV loads suitable for VGI participation represent nearly 400 GWh of storage capacity in 2030 and more than 1500 GWh in 20501 Light-duty vehicle electricity demand in California 1. This analysis assumes average EV pack size increases to 80 KWh by 2030
3. Modeling results
page 20 • The least-cost, high-electrification pathway in this analysis relies on expanded renewables for low- carbon electricity and targeted clean fuels to achieve economy wide net-zero emissions in CA by 2045. • With annual electricity demand more than doubling in CA by 2045, the base scenario relies on renewables for energy production and a mix of gas power plants with clean fuels, storage and large-scale flexible loads to maintain reliability • Enabling 50% V1G in the base scenario reduces energy system costs by $4 billion per year in 2045 (over $500 per vehicle), representing a 1.7% reduction of California’s total energy system cost • The scenario with 50% V2G offers even greater savings, lowering system costs by $11 billion per year, which equates to nearly $1,200 per vehicle in 2045 • V2G enables higher savings by occasionally discharging from vehicles to support system reliability and avoiding reliability-related costs • While V2G does avoid some battery storage investments, V2G has a limited roll in shifting solar production from the middle of the day to the evening, it principally creates value by avoiding electric delivery related costs and supporting reliability for the bulk system • While all levels of VGI provide savings by avoiding distribution costs associated with higher peak demand and reducing capacity build, higher shares of V2G than V1G generate larger savings Summary of modeling insights
Modeling results: Decarbonized California Energy System
page 22 • The base scenario reflects the state’s vehicle and emission reduction policies, and final energy demand reflects economy-wide electrification for California. • Electricity’s share of final energy increases from 60% by 2050. • Electrification supports decarbonization for California by replacing fuel combustion with electricity, which is a lower-cost clean energy carrier, and for many end uses, including passenger vehicles, it also improves efficiency. • The base scenario is adapted from the central scenario of EER’s 2024 Annual Decarbonization Perspective, reflecting a nationwide high-electrification demand-side scenario. The base scenario reflects California shifting toward electricity and away from other fuels
page 23 • Growth in renewables meets the growing demand for electricity and displaces gas generation. • Solar capacity and generation increase significantly, up nearly 150% in 2045 compared to 2024. With the State’s abundant solar resource, large solar deployments supported by utility scale storage is economic in the base case. • Offshore wind and geothermal generation both increase significantly after 2030, reaching 22GW and 16GW respectively in 2045. • Gas generation as a share of total generation falls rapidly, but total capacity declines gradually to help meet the reliability needs of the system with residential peak loads increasing 120% by 2050 and bulk system peak demand increasing by 35%. Storage, geothermal, and transmission also play an important role in reliability. • As with the other assumptions behind the base scenario, the main cost and build rate assumptions that shape the scenario are taken from the Central scenario of the 2024 Annual Decarbonization Perspective.1 A major expansion of renewables meet the growing electricity demand in the state 1. The only change from 2024 ADP assumptions are modifications to geothermal assumptions for this analysis to better align the least-cost portfolio with the most recent long-term planning process results. See the appendix for more details on the geothermal assumptions.
page 24 • As the electrification of vehicles, buildings, and some industrial applications increases, the remaining fuel demand declines while the blending of low-carbon biofuels and synthetic e- fuels (fuels derived from captured carbon and zero-emission hydrogen) increases. • The state’s emission cap results in high levels of clean fuel blending, including biofuels into pipeline gas, which is often at the end of the fuel decarbonization supply curve given the marginal cost of displacing natural gas as compared to other fossil fuels that have higher emissions per unit energy. • Despite growing demand for aviation, jet fuel demand remains flat due to efficiency measures in aviation. Jet fuel rapidly increases the blending level, with e-fuels eventually displacing biofuels as the marginal value of biomass feedstocks increases with more stringent emission limits. • The role of e-fuels is influenced by several factors, including excess renewable generation that can potentially support electrolysis for hydrogen production, the relative costs of capturing carbon, and the economics of biofuel alternatives. E-fuel deployment rises as the scale of renewable generation increases, and more binding emission limits change the economics of where biofuels are used. The remaining demand for fuels is increasingly met by low-carbon, drop-in replacements
page 25 • The base scenario achieves net-zero emission in California by 2045, where sequestration, including land sinks, offsets the remaining emissions, primarily non-CO2 greenhouse gases. • Electrification and greatly expanded clean electricity generation lowers fuel consumption, enabling emission reductions to meet the state’s emission goals. • Negative emissions technologies, like biomass gasification for hydrogen production with carbon capture and storage, enable net-zero in 2045 and 2050 as energy and industry CO2 emissions approach zero, and the remaining emissions have limited or no abatement options. Electrification, large-scale renewable deployment, and clean fuels achieve net-zero emissions by 2045
Modeling results: Savings and impact of VGI
page 27 • For the set of scenarios where half of the eligible light-duty EV load participates in VGI, 2045 savings for CA range from $4B/yr to $10B/yr ($503- $1,177 per vehicle) • These savings represent 3.2% to 4.4% of total energy system costs for the state. • Savings increase with higher levels of V2G participation for a given level of VGI. V2G captures the benefits of V1G-optimized charging and the ability to discharge. • Going from the 50% V1G scenario to the 50% V2G more than doubles savings Smart charging half of eligible VGI load saves $500 per vehicle in 2045, V2G yields greater savings
page 28 • VGI-driven savings come from lowering electricity delivery costs (e.g., avoided distribution costs), reductions in storage and thermal capacity as well as lower investments in renewables. • The flexibility from optimal charging displaces the need for battery storage along with some thermal capacity. This true smart charging is responsive to renewable energy supply, shifting consumption to when there is abundant supply and changing the composition of renewable build in each scenario. • As the relative share of V2G increases, savings increase as more costs from capacity and electric distribution are avoided. • The savings from avoiding electricity delivery costs (reducing peak demand at the feeder level) grow the quickly as V2G share increases with the ability to discharge being used in a targeted manner to lower peak demand. • In addition to reducing feeder peaks, the ability of VGI to discharge changes how reliability is met in a least-cost system, with V2G displacing some reliability-driven investments like long-duration storage. • Renewable costs vary as V2G increases, as the economic mix of renewable generation transitions to a shape that better works with the flexibility and reliability provided by V2G increasing savings for some scenarios as compared to 50% V1G. • Costs associated with fuel production, which by 2045 are almost entirely decarbonized, rise with higher levels of V2G. More V2G changes the reliability paradigm, relying less on electrolysis as a large-scale flexibility resource, shifting clean fuel production away from e-fuels. Lower e-fuel production avoids some direct air- capture investments, but increases fuel-related costs overall as more biofuels are deployed. Avoided distribution, storage, renewable and thermal capacity investments drive savings
page 29 • Feeder peak demand is the key driver of electricity distribution delivery costs, so lowering peak demand avoids delivery costs • Electrification significantly increases electricity demand in the state, and the base scenario increases residential peak loads between 2024 and 2045 by more than 50% in both northern California (10 GW increasing to 16 GW) and southern California (16 GW increasing to 24 GW). The commercial feeder shows much smaller increases in peak load, and new industrial loads also drive significant growth. • VGI helps to offset the growth in peak demand, with the 50% VGI scenarios reducing residential peak demand by 10% and commercial feeder peaks by 3.5% • VGI flexibility represents a small share of industrial feeder load and reduces less than 1% of demand on the feeder • Increasing levels of V2G results in greater peak reductions for all feeders • Targeted V2G discharge can help reduce peak demand, enabling greater savings VGI reduces electricity delivery costs by lowering peaks on residential and commercial feeders
page 30 • VGI directly competes with high-cost, long-duration storage. True-smart charging shifts demand away from periods that would have relied on long-duration storage to meet demand, and V2G is able to displace more long- duration storage. • Some amount of battery storage plays a complementary role to VGI, with high V1G scenarios adding a small amount of battery storage to enable savings from avoiding other costs. Increasing levels of V2G capabilities displaces battery storage and long-duration storage. • Increasing shares of V2G can displace the role of battery storage of discharging in the evenings on key reliability days, but it’s not economical to discharge V2G daily. • Charging flexibility from VGI changes the economic mix for renewables, trading some solar and geothermal capacity for additional wind capacity, lowering costs. • Smart charging plays a critical role in better-aligning load with the least-cost renewable mix. It should be noted that small changes in projected renewable costs could change the economic mix of renewable build. • While V1G reduces thermal and long-duration storage build, higher V2G levels displace even more resources with a reliability role. • Thermal capacity decreases with higher shares of V2G as the ability to selectively discharge vehicles changes the economics of reliability, reducing the need for thermal capacity as a reliability resource. • Long-duration storage is a costly means of providing reliability, and high levels of V2G reduce the deployment of this resource. VGI’s flexibility decreases storage and thermal build, changing the economic mix of renewables
page 31 • VGI transforms the LDV charging shape into a flexible resource that responds to system needs on an hourly basis. • Optimized charging works on each feeder to respond to hourly needs, shifting load to avoid feeder peaks and to align with renewable generation. • This contrasts with bulk system battery storage that is deployed to meet bulk system needs, principally shifting solar production to later in the day, and shows less variability in behavior than VGI charging. • Discharging from V2G is infrequent, only supporting reliability needs on key days and hours rather than shifting solar production to later in the day. • While V2G avoids some level of battery build, the remaining bulk system storage complements V2G’s behavior, with each providing different forms of flexibility that support a reliable, least-cost system. • All scenarios with V2G, including the 80% V2G scenario, show similar behavior of discharge isolated to key reliability periods. Both VGI charging and discharge vary daily in response to system conditions
page 32 • To explore the robustness of the value of occasional V2G discharge, this analysis included a sensitivity on the 50% V2G scenario exploring the technical potential of V2G, which relaxes the constraints on discharging • Nearly four-fifths of the total V2G energy discharged in the 50% V2G scenario occurs during the 60 days with the greatest daily discharge, where select hours use more than half the available discharge capability. • Occasional discharge from V2G is driven by a number of factors, including the assumptions that constrain V2G behavior in this analysis, like variable O&M of $100/MWh and state-of-charge penalty are key assumptions that limit how often V2G discharges. • This V2G technical potential sensitivity reduces the variable O&M to $1/MWh, and removes the state-of-charge penalty except for when state-of-charge falls below 30%. • The V2G technical potential sensitivity increases V2G discharge by a factor of almost four but only increases the savings of the 50% V2G scenario by less than 10%. • The V2G discharge captures more than 90% of the benefits from the technical potential sensitivity with discharge that is equivalent to adding 600 miles per year (compared to more than 2,000 miles per year for the technical potential scenario). Occasional V2G discharging captures 90% of the potential value of V2G participation
page 33 • Solar generation plays a critical role in California’s decarbonized energy system by 2045. The ability of VGI to charge and discharge like utility-scale battery storage could potentially increase savings by avoiding storage. However, most of the VGI is on the residential feeder, which has lower charge and discharge capability (fewer vehicles available) in the middle of the day. • To explore if the envelope for maximum charge and discharge was limiting VGI’s ability to shift solar production from the middle of the day to more valuable periods, EER developed a sensitivity with higher potential charge and discharge throughout the day (‘high pmax’ for short), particularly in the middle of the day. • The high pmax sensitivity on the 50% V2G scenario slightly increases savings in 2045, increasing savings from $10.7B to $11.4B (an increase of 6.6%). • While battery storage capacity is lower in the high pmax sensitivity (a decrease of less than 1%) it accounts for less than 5% of the incremental savings for the sensitivity. The key factors in sensitivities higher savings are greater peak reduction and lower renewable capacity build. The higher charge capacity in the middle of the day improves solar utilization and lowers solar build, and the greater discharge capacity increases the annual V2G discharge by more than 45% to lower distribution costs. • The higher charge and discharge sensitivity shows limited additional savings, with most of the incremental savings coming from avoided distribution costs. This indicates that the charge and discharge constraints in the 50% V2G scenario already capture nearly all bulk-system savings. More ability to charge in the middle of the day only modestly increases savings from VGI
page 34 • Looking at the full range of scenario results, comparing the share of V1G (x-axis) against the share of V2G (y-axis), shows higher levels of V2G results in greater net savings • At 20% VGI, the savings for all V2G scenarios are 400% higher than the V1G-only savings. • At higher levels of VGI, V2G only scenarios represent a smaller increase in savings over V1G-only scenarios. • The same dynamics of how VGI creates savings at 50% of load also applies at 20% and 80% • Most of the savings come from avoided delivery costs, and higher relative shares of V2G unlock more avoided capacity savings as V2G discharge enables a lower-cost approach to a reliable electricity system The full sweep of scenarios show the incremental value of V2G is highest at lower levels of VGI
page 35 • The full results show that higher levels of V2G (moving further right on the x-axis in this figure) yield smaller incremental savings than lower levels of V2G • At 80% VGI, additions of V2G beyond 25% of all VGI represents small incremental savings • Increasing the total level of VGI also shows diminishing returns • V2G-only scenarios show declining savings per unit of participation as the level of VGI increases, dropping by nearly 70% when moving from 20% to 50% participation and more than 70% going from 50% to 80% participation. • V1G-only scenarios show much smaller declines in savings per unit of participation, decreasing by 10% when moving from 20% to 50% V1G participation and decreasing by 15% going from 50% to 80% V1G participation V2G shows significant diminishing returns, flattening its impact on savings at higher levels
page 36 • The maximum savings per vehicle are for the 20% V2G-only scenario at over $1,300 per vehicle • V2G is the most valuable form of VGI for vehicles, and greater numbers of vehicles participating in VGI increases total savings but reduces savings per vehicle. • As with total savings, increasing shares of V2G at a given level of VGI grows savings per vehicle, representing 185%-250% more than V1G only depending on the total amount of VGI • As with net savings, higher levels of V2G show diminishing returns, particularly for any scenario where V2G is more than 25% of VGI eligible load Savings per vehicle decline with higher shares of V1G and with higher total VGI
4. Looking Forward
page 38 • Future improvements in data, VGI analysis, and battery technology will be key factors in scaling VGI participation and expanding benefits to the energy system and individuals. • Implications for ramping up VGI deployment: • Improvements in data quality and refinements to the analytical approach will be crucial for the long-term deployment of VGI, including developing programs and processes needed for VGI to realize system-level benefits. • The full range of benefits of VGI will be a key determinant of adoption, particularly direct customer benefits, which were not considered in this analysis. • Advances in battery technology for light-duty electric vehicles could change the economics of V2G participation with lower battery degradation costs. Ramping up the deployment of VGI
page 39 • Accurate data for actual EV charging profiles will be essential for improving estimates of VGI savings for the energy system. The charging profile defines the baseline for measuring VGI savings. Current data sources rely on outdated data sets and do not incorporate aggregated meter-level data that is becoming more available for planning agencies. • More granular trip data combined with charger location data are important supplements to improved charging shape data that can inform the charge and discharge capabilities of VGI participants. • VGI programs will need to account for how responsive participants are to calls for different charging or discharging behaviors. Future analyses of VGI will benefit from data on real-world responsiveness rates, and this data will also be key for VGI program design and ongoing planning. • Similarly, data of driver preferences (e.g., level of charge, willingness to pay when extra charging is needed) will be crucial for understanding customer-level economics of participating in VGI. • Analysis with finer geographic resolution, including the ability to represent discharging and charging across locations and feeders, are needed to identify high-value areas for early VGI deployment and developing a road map for achieving VGI at large scale. Better data and future analytical improvements can support planning for scaling VGI
page 40 • The analysis for this report was limited to considering energy system benefits and does not capture the wide range of customer benefits enabled by the infrastructure needed to support true-smart charging and V2G. • Customer benefits related to bulk system savings and distribution-level avoided capital costs will be shaped by VGI program design, which will need a means of passing savings back to the participating vehicle owners. This aspect of program design will be a critical consideration for scaling VGI. • A key form of savings to be mediated through VGI program design is the savings from true-smart charging, which attempts to move charging to periods with low-cost electricity supply, potentially utilizing renewable energy that might be curtailed in the absence of V1G. V1G will lower charging costs for the system as well as individual consumers. • V2G participation may enable direct customer benefits of backup power. The resiliency benefit of V2G enabling infrastructure could provide backup power from the EV’s battery, could directly benefit for some customers, particularly with the increasing prevalence of Public Safety Power Shutoffs in various parts of California. Direct customer benefits will be a significant factor in VGI adoption
page 41 • As of 2025, almost all battery electric vehicles (BEVs) use one of two lithium battery chemistries: • Li-NCx - lithium nickel cobalt oxides, including nickel-manganese-cobalt and nickel-colbat-aluminum, are the current global standard and are used longer range EVs. This battery chemistry has higher energy density (longer vehicle range) but is more expensive and is projected to have a shorter lifespan (faster degradation with cycling). • LFP - Lithium iron phosphate batteries have a shorter range but are cheaper and potentially more durable. LFP has become the leading technology in China but has limited market penetration in California. • Solid state batteries, along with many other chemistries, are under development and are expected to eventually produce batteries that are high density, safe, cheap, and durable. • The durability of future EV batteries will matter for V2G deployment because it impacts the willingness of customers to participate. The term “million-mile battery” was introduced in a 2019 publication. • Today it is common to avoid charging past 80% with Li-NMC (reflected in our assumptions for customer participation cost), but if batteries could be charge/discharged in the 80-100% with little impact, sensitivity analysis shows an increase in the value of V2G by 6%. New battery technologies could enable more valuable V2G participation
page 42 Next steps for California stakeholders Utilities Regulators Investors Policy Makers VGI can lower distribution-level peak demand. This study found more than a 25% reduction for residential customers in 2045, controlling distribution costs and mitigating rate increases that would occur without VGI. To unlock VGI savings, it must be fully incorporated into resource and distribution planning. Regulators can shape early deployment and evaluation efforts essential for achieving deployment at scale. The long-run value of V2G comes from displacing reliability resources, not shifting solar production daily. Successful early investments will build VGI products that tie together software, program structure, and hardware that can enable vehicles to provide reliability for the distribution and bulk system. VGI is vital for the long-term success of California’s energy policy by controlling costs. Early investments in the technology through expanded pilots, additional incentives, and R&D will pay dividends for California ratepayers.
Appendix A: Methodology
page 45 EER analytical tools used in this study EnergyPATHWAYS (EP) is our demand-side stock- rollover accounting model that produces scenarios based on exogenous service-demand and sale shares RIO is a supply-side macro-energy model that finds the lowest cost investment and operations plan with best-in-class temporal and spatial granularity 2021 Energy System Annual End-Use Energy Demand Hourly Load Shape Deep decarbonization pathway with electrification
page 46 • Capacity expansion tool producing cost-optimal resource portfolios across the electric and fuels sectors • Least-cost energy supply mix to achieve emissions targets • Simulates hourly electricity operations and annual investment decisions • Electricity and fuels are co-optimized to identify sector coupling opportunities • Example: production of hydrogen from electrolysis Supply-side modeling Electricity Pipeline Gas Jet Fuel Diesel Fuel Gasoline Fuel Hydrogen Co-optimized energy supply
page 47 • The modeling assumptions for this analysis align with ADP 2024 except for where we’ve made revisions to explore light-duty BEV VGI in the context of California. To focus our analysis, the following study assumptions differ from ADP 2024. • The model incorporates numerous updates related to representing VGI, as described earlier in the report • Both V1G and V2G representations incur a penalty for deviating from a SOC of 80%. • Optimized charging is constrained by a maximum charging shape, which reflects travel patterns on different feeders. This charging envelope is based on the ‘slow as possible’ charging shape from the EVI-Pro tool. In 2045, peak charge capacity varies by feeder, but ranges between 1.3kW and 2kW per participating vehicle. Peak charge capacity is derived from the annual peak of the ‘slow as possible’ charging shape for the modeled CA zones. • V2G discharging is similarly constrained by a discharging shape. This ‘discharge envelope’ is also adapted from the EVI-Pro tool’s ‘slow as possible’ charging shape. Peak discharge per vehicle is assumed to be higher than peak charge, over 5kW per vehicle on the residential feeder. Peak discharge is defined by the assumed average EVSE capacity per vehicle (assumed 9.6 kW), derating for an assumed 1.5 enrolled vehicles per charger, and assuming 20% of vehicles are unavailable during a given discharge event (e.g., not at home and plugged in). • V2G discharging has a round-trip efficiency of 80%1 and also has variable operations and maintenance costs, which are described on the next slide. • Interzonal transmission expansion is fixed to the level of expansion in the base scenario, so VGI cannot increase or decrease transmission build. • ADP 2024 included significantly more enhanced geothermal potential than previous California long-term energy modeling analyses, so the ADP 2024 geothermal potential was reduced, assuming only 25% of it is developable, to better reflect the long-term resource mix the state is currently planning around. Key modeling assumptions beyond ADP 2024 1. https://op.europa.eu/en/publication-detail/-/publication/ceaddb39-7763-11eb-9ac9-01aa75ed71a1/language-en
page 48 • Discharging V2G creates additional degradation on a vehicle's battery, similar to increasing annual mileage • In order to capture the cost of additional wear from discharging, V2G is modeled with a variable O&M cost that approximates the implied $/MWh cost of shortening the duration of a vehicle's battery warranty • A shorter warranty represents a higher present cost • The difference in the present cost between the warranty without V2G discharge and with a shorter warranty from V2G discharge represents the amount that would make the owner indifferent to a shorter warranty • The calculations in the table show one set of assumptions to estimate an implied $/MWh variable O&M • Numerous assumptions go into the implied VOM calculation, including the assumed energy discharged for V2G from a vehicle during its warranty. • For this analysis, we assume $100/MWh for V2G variable O&M, which is indicative of the range of results given varied assumptions on efficiency, pack cost, and discount rate. Estimating variable O&M cost for V2G discharge Example assumptions Value Warranty miles 100,000 a EV efficiency (miles per kwh) 3.3 b MWh covered by warranty without V2G 30.3 a/b/1000 Pack replacement cost $10,000 c Annual mileage 12,000 d Assumed discount rate 6% e Warranty years without V2G 8.3 f = a / b Present cost of warranty without V2G $6,153 g = c * (1 + e)^-f Warranty years if 2 MWh are discharged for V2G 7.8 h = (a – 2000kWh * b)/d Present cost of warranty with 2 MWh of V2G discharge $6,354 i = c * (1 + e)^-h Implied $/MWh value 100.2 (i – g) / 2 MWh
Appendix B: Supplemental results
page 50 Cost savings by category for the full sweep of scenarios
page 51 Electricity supply and demand on a sample day with V2G discharge
page 52 Average state of charge by hour for VGI on different feeders